Reducing CO2 emissions is one of the most important global tasks of our time. In industry in particular, measures to minimise carbon dioxide emissions are urgently needed.
In this blog post, we will focus on comparing the CO2 emissions of conventional and additive manufacturing processes when using precious metals.
The study
The study „Development of a model to determine the life cycle assessment and carbon footprint of precious metals“ was carried out by the Institute for Industrial Ecology (INEC) at Pforzheim University on behalf of C.HAFNER.
The aim of this study was to analyse, measure and compare the CO2 emissions of different manufacturing processes for identical products. To this end, two representative products from C.HAFNER’s product portfolio were selected that can be manufactured using both conventional and additive manufacturing methods. As both manufacturing processes are used in C.HAFNER’s production practice, they provide valuable data for the analysis. The selection of products from the C.HAFNER portfolio was carefully chosen to ensure appropriate representation:
- a watch case made of an 18-carat gold alloy, as a representative product for the luxury goods industry
- an electrode head made of Pt80Ir20, as a representative technical functional part for the technical industry
Conventional production with precious metals
Conventional manufacturing includes traditional processes for the production of precious metal products such as casting, forming and machining. Several energy-intensive steps are required in production, including melting the metal or mechanical processing.
Additive manufacturing with precious metals
Additive manufacturing enables the production of components by applying and fusing material in layers. There are two basic additive manufacturing principles. In sinter-based processes such as binder jetting, a mixture of binder and metal powder is first plotted and then thermally transferred into the finished metal part. In direct methods such as the laser metal fusion used here, the metal powder is applied in layers without additives and fused into finished components using a laser. Both processes offer a number of advantages, including lower material input quantities or greater design freedom. But what do you believe about CO2 emissions?
Additive and conventional manufacturing processes: Carbon footprint of the starting material
The basis for calculating the total CO2 emissions of the entire manufacturing process of a component is the carbon footprint (CF) of the individual materials. The calculation is based on a model for determining the life cycle assessment and carbon footprint of precious metals and the resulting CF of the pure metals used and process1.
CO2 emissions of both manufacturing processes in comparison
As the reference products at C.HAFNER could be manufactured both conventionally and additively, energy and mass flows of the process chains for these products were determined using real production data and converted into CO2 equivalents (CO2e) on the basis of the above-mentioned Umberto model.
Comparison of the CO2-equivalent shares of electrode production in platinum
In the case of conventionally manufactured electrodes, the CF totals 3.174 kg CO2e per part of 4.4 g each. The largest shares of the CF, at approx. 0.7 kg CO2e each, are attributable to the fine metals, the energy required in production, and the chemicals and energy required in the recovery of production waste containing precious metals (see Fig. 1).
In additive manufacturing, the proportion of fine metals in the CF is still the same, but the energy and material requirements for the processes are significantly lower. In total, electrodes manufactured in this way only cause a CO2 equivalent of 2.234 kg CO2e per part of 4.4 g each.
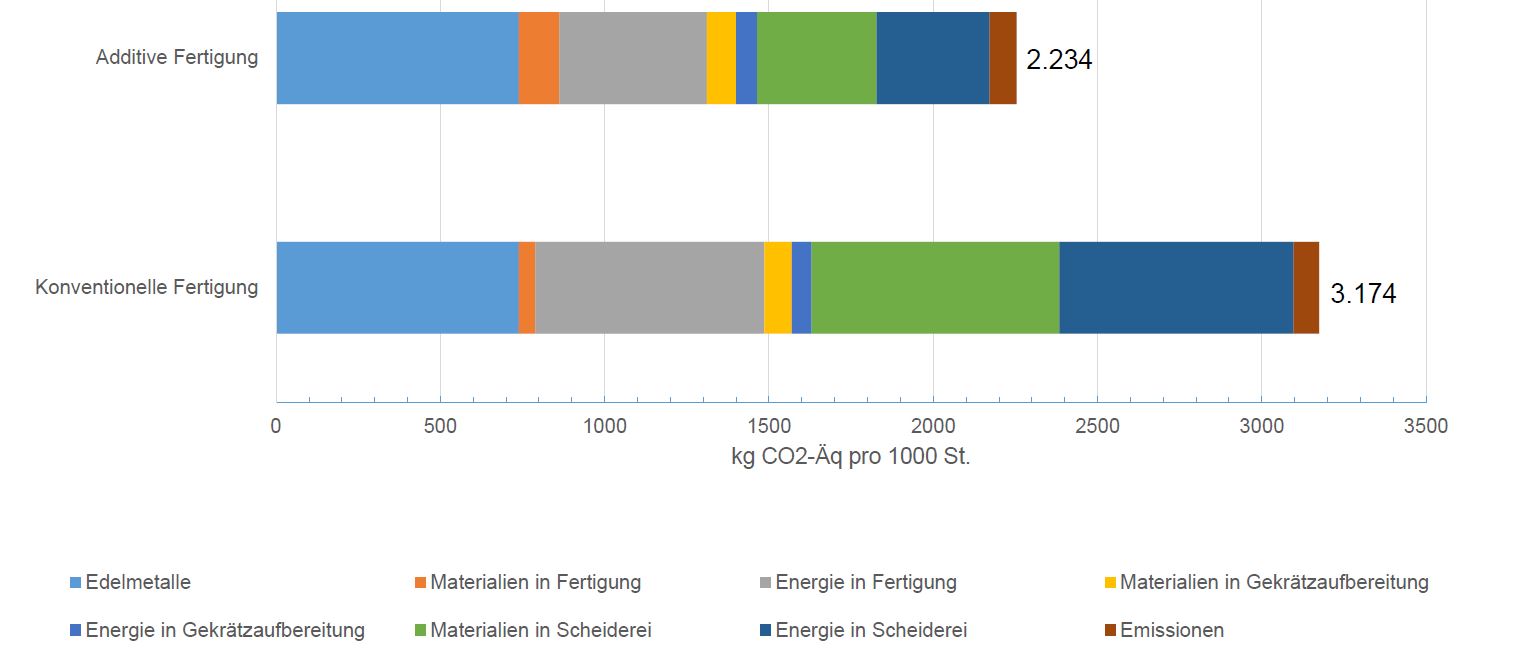
Fig. 1 Comparison of the carbon footprint (1000 electrodes), please click on the diagram to enlarge
Comparison of the CO2-equivalent shares of watch case production in gold
The differences in favour of the additive route become even clearer in watch case production. A conventionally manufactured watch case causes a CF of 30 kg C CO2e per part of 41 g each, with the recycling costs for material waste in particular accounting for a large proportion. In comparison, the watch case based on additive manufacturing has a significantly lower footprint of just under 14 kg CO2e per 41 g part, mainly caused by the energy required in production with significantly lower fine metal requirements and expenditure for precious metal recovery from returned materials.
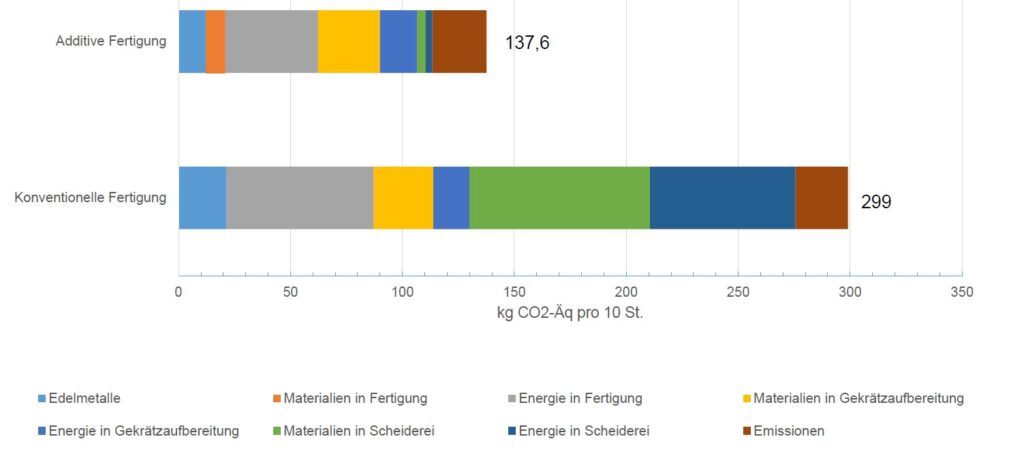
Fig. 2 Comparison of the carbon footprint (10 watch cases), please click on the diagram to enlarge
Result of the study: How do the CO2 footprints compare?
The CO2 footprint of the exemplary electrode made of Pt80Ir20 can be reduced by 31% through additive manufacturing (2.2 vs. 3.2 kg CO2e per part at 4.4 g). In the case of the watch case made of 18k gold, the CO2 footprint is even over 50% smaller (14 vs. 30 kg CO2e per part at 41 g).
The biggest influencing factor is the amount of returned scrap and the effort involved in the separation process. The lower amount of material used and thus the volume of material to be separated are a significant advantage of 3D printing technology, which enables production very close to the final mould compared to conventional methods. As a result, the capital expenditure in the process chain for additively manufactured products is also significantly lower.
Conclusion
The CO2e savings potential through additive manufacturing with precious metals is one third to more than half in the two examples analysed. The potential of other components can be further increased by utilising additional design freedoms and possibilities, particularly for further weight reduction through additive manufacturing. This shows that additive manufacturing has significant potential for CO2 savings compared to conventional manufacturing and can therefore make an important contribution on the road to climate neutrality.
Sources:
1 Schmidt, M., Fritz, B.: Entwicklung eines Modells zur Ermittlung der Ökobilanz und des Carbon Footprints von Edelmetallen. Bericht des Instituts für Industrial Ecology (INEC) an der Hochschule Pforzheim Im Auftrag von C.HAFNER GmbH + Co. KG. erweiterte Fassung nach Review durch Rolf Frischknecht, treeze Ltd., fair life cycle thinking, Mai 2022.